Future strategy of surgical simulations in video-assisted thoracic surgery
Introduction
Video-assisted thoracic surgery (VATS) has come to be the primary operative procedure in thoracic surgery and is gradually substituting open thoracotomy as the method of choice for the treatment of lung cancer, metastatic lung tumors, mediastinum tumors, and benign lung diseases (1-7). Established evidence of several advantages in VATS presents faster operative recovery, fewer complications, and quicker return to daily life preserving quality of life without interfering with oncological and survival outcomes when compared to thoracotomy (8-13). As VATS has acquired widespread favor with the rapid development of techniques and instrumentation, indications for VATS have further expanded. However, many medical facilities have yet to adopt the thoracoscopic approach for anatomical lung resections because learning and mastering this technique is a real challenge due to the technical demand of being get used to a two-dimensional surgical field being viewed on monitors and the use of long specialized devices. Moreover, the new variant, uniportal VATS, also emerges as a one-step-forward approach toward the same minimally invasive direction (14-16).
In terms of building VATS educational platforms today, standardization of the training programs that is based on risk-free based teaching strategy is essential and would involve structural and functional alterations. Unstructured traditional apprenticeship of unlimited duration used to be the most common trend available for surgical residents wanting to acquire major surgical skills and dexterity with supervision tailored to their needs. Although this confers experience in dealing with a wide range of operative approaches and complications from basic skills such as knot-tying or suturing to the execution of fearsome steps such as controlling bleeding by unexpected vessel injury on trainees over many years, these types of training should be usually leant only inside the operating room. Duty-hour restrictions and decreased clinical exposure of trainees in many countries and recent radical changes in the prevalence of minimally invasive surgery produce new challenges and might require additional resources without causing a deterioration of required surgical residency’s experience (17-19). Moreover, an increased chance of legal disputes and strong ethical imperatives also attribute reduced surgical training opportunities on patients to young surgeons (20,21). More efficient educational curriculums than the traditional apprenticeship design is therefore warranted for novice surgeons to improve their cognitive and procedural skills in a stepwise manner. Today, surgical simulations have a pivotal role in increased patient safety and reduction of the risk of surgical errors as a desired part of the training model for not only residents or fellow surgeons but also experienced consultant who need to acquire new techniques (22-30).
When thoracic surgeons work with simulators that are used for training individuals by imitating situations they encounter in real life, there are generally two different categories of surgeons requiring skills of thoracoscopic procedure (or already reaching a higher proficiency level to accomplish VATS) who must be considered in the community; (I) surgeons who had experienced a period of transition from open thoracotomy to VATS, and (II) surgeons being trained after the exponential development of VATS. Surgical simulations work differently between these two cohorts according to their surgical skills and experience. Majority of the current leaders and pioneers with VATS expertise are the former and had seen the whole evolutionary process from thoracotomy to VATS. They were usually self-taught and gained experience and confidence over the years. On the other hand, most of current young surgeons are the latter and this new generation have usually initiated their surgical specialty trained mostly in thoracoscopic or laparoscopic procedures under guided supervision whereas they have not so many experiences of open chest surgery. The evolution of advanced technologies including a variety of simulation programs such as human cadavers, live animals, black-boxes, and three-dimensional (3D) virtual reality simulators can compensate their limited-operating room-based training opportunities and inexperience that may cause intraoperative complications and aid them in the acquisition of necessary thoracoscopic skills in a brief time (26-29,31,32).
Here, we will discuss the types of simulators currently available focusing on the desired programs to allow the coming generation to master VATS lobectomy with a shorted learning curve and future strategy of surgical simulations in VATS.
Advantages of simulation-based training
Not only surgeons but also other professions such as pilots and soldiers practice and learn in real-time interactive simulations. Pilots log hundreds of hours learning to fly new planes in simulators before they ever enter the real plane, so that they already know how to operate it in a wide range of conditions on taking the real plain up. Likewise, surgical simulation is a very important aspect of training in learning counterintuitive techniques and promote repetitive practices in a setting that forgives failure before doing operation for real patients. A key question here is whether thoracoscopic skills learnt on simulators translate to improve actual performance on patients and make the learning curve of VATS lobectomies shorter.
Supportive evidences from many studies suggested that a variety of simulation methods and dedicated dry and wet labs help to enhance psychomotor and judgement skills, hand-eye coordination, and ambidextrous surgery (33). An ex vivo model such as porcine heart-lung block filled with ketchup offers a low cost and high-fidelity simulation if the tissue box is prepared with bench-top models (27,28). The use of live animals such as swine proved to improve greatly the learning of basic and advanced surgical procedures (30). Live animals share many of the same features as human surgeries and provide trainees with a wide range of VATS procedural elements (30,34). Simulated environment provided by live animals is superior to other kinds of simulators in the context of the accuracy and reality. However, they are limited by the cost, single use of animal tissue, and some ethical concerns.
Having less anatomical accuracy than live animals and cadavers, another low-fidelity model such as black-box simulator allow quick and repetitive simulation of a specific skills enable to mastery of individual techniques (28,29,35). Modern 3D virtual reality simulators also offer high-fidelity and create realistic environment that capture minute anatomical details with high accuracy (31). Virtual simulator-based training developed for laparoscopy showed that the performance level of novice surgeons was increased to that of intermediately experienced laparoscopist in a randomized controlled trial (36). Jensen et al. reported that when making comparisons between virtual reality simulation and black-boxes in overall skill acquisition after video-assisted procedure completion, surprisingly the black-box group was significantly faster than the virtual-reality group (29). The authors also demonstrated a commercially available virtual reality simulator for practicing VATS lobectomy showing good content validity in another study (31). The amount of these dry lab exercises is unlimited, and the cost is small once the systems are installed. Refined virtual reality simulators enable technical performance to be recorded, measured, and used for feedback.
Recently, 3D-printed Biotexture wet lung models for surgical training were introduced as a valid alternative to the use of animals (Fasotec, Chiba; Figure 1). Texture of human tissues are reproduced to enable surgical trainee enhance practicing. Being installed inside the thoracic cavity simulator, the wet model offers the realistic environment for VATS training (Figure 2). Such a new advanced surgical simulator provides appropriate levels of challenge and instruction.
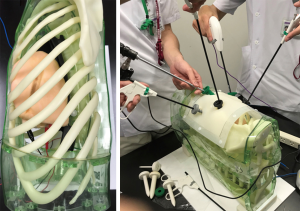
The strength of surgical simulations in VATS as an adjunct rather than an alternative to clinical experience (37,38). The technological evolution has paralleled the evolution of surgical simulators, which offers obvious training and educational benefits.
VATS simulation programs of the future for the new generation
A key challenge is to lay the formal and structured VATS training base incorporating available surgical simulations to safe VATS lobectomy completion for the next generation of thoracic surgeons. As each operation has a sequence of steps of increasing difficulty, they should focus on completing each thoracoscopic procedural element in a step wise fashion by practicing basic parts to difficult parts no matter what the level of experience of the trainees. Even though they have a wider range of surgical techniques to learn and inevitably have less experience in certain techniques including open chest procedures than the forebears had, standardized VATS lobectomy with preserved quality has to be completed without compromising patient safety, acceptable operative time, and minimum blood loss through an appropriate supervision. Surgical simulations are supposed to contribute to the shorter learning curve of VATS lobectomies and provide an adequate return on the investment of both finances and time. Here are a set of recommendations for a surgeon embarking on structured VATS lobectomy program with taking advantage of surgical simulations even though many different better approaches to VATS lobectomy have been existed:
- Dry lab exercises (virtual reality simulators and black boxes) and ex vivo model (porcine heart-lung blocks);
- 3D printed Biotexture model;
- Wet lab exercise (live animals) and VATS minor procedures;
- The introduction of VATS lobectomy in a stepwise fashion and 3D image surgical simulation.
Once trainees begin with a new step, that has to be performed repeatedly within a short period of time. Otherwise it will take a long time to complete a learning curve. On every step, surgeons have opportunities to review the video with their performance over and over, preferably along with senior surgeons in order to critically analyze it.
As an initial step, students and starters receive training on thoracoscopic box and virtual reality simulators as well as using the porcine blocks. Black-boxes require them to operate within the closed environment containing scopes that allow them to check their own movements (29,35). Virtual reality simulators also offer real-time haptic feedback to trainees about their performance within the simulation (31). The porcine block combined with bench-top synthetic model is a highly useful and inexpensive simulator with high fidelity (27,39). The efficacy of these dry lab exercises and ex vivo training in improving surgical skills in a cost-effective manner has been validated by multiple studies (40-42).
Once trainees attain significant proficiency in basic simulated thoracoscopic tasks over a relatively short period, the next step is to exploit high fidelity models such as 3D-printed wet models, live animals, and cadavers to make their acquired skills more advanced and improved. Although some ethical concerns have arisen over the use of live animals and cadavers as simulators depending on countries, VATS courses with wet labs can have a different educational focus according to the experience of the group. In the course of acquiring advanced skills using the simulation models, surgeons have to accumulate an exhaustive knowledge of their anatomical characteristics, which can foster the crucial point of views for grasping detailed surgical anatomies and 3D relationships of hilar structures on real patients. In addition to the wet lab exercises, performing a lot of minor VATS procedures such as pneumothorax operations, pleural biopsies, division of pleural adhesions, and wedge resection can be better options at this level while considering the differences in anatomy between animal’s and human’s. At the same time, trainees at this level need to learn the principle of VATS scopist until they can show reproducible operative fields whenever operators ask for.
After the completion of “warm-up” period of the trainees, the introduction of VATS lobectomy procedures can be provided in a stepwise fashion; port replacements first, then dividing the pulmonary ligament, dissection of pulmonary vein, artery, and bronchus, and lymphadenectomy. Even if surgeons are get used to perform thoracoscopic procedures, surgical simulations still matter. 3D imaging techniques has come a long way since its introduction in the field of thoracic surgery. Many studies reported that surgical simulations for the assessment of chest anatomy using as variety of 3D imaging modalities are highly beneficial for the safe and efficient performance of VATS anatomical resection and for further understanding of the surgical anatomy (43-46). Moreover, preoperative understanding the anatomy of the lung and many anatomical variations beforehand by 3D images also make the learning curve shorter. Regardless of the experience of VATS lobectomies, it is crucial for surgeons to perform surgical simulation for each case.
Surgical simulators of the future are considered to move forward to “patient-specific simulations” that is similar to the underlying concept of precision medicine, in which health care is individually tailored on the basis of a person’s genetics, lifestyle, and environment. If digital imaging and communications in medicine (DICOM) data that have high resolution computed tomography of a patient are transferred to virtual reality simulation modalities, then a surgeon can practice VATS anatomical resection repeatedly with reusable patient specific virtual reality lung structures. These types of simulators have already been used in other areas of operations (47-49). Patient-specific lung virtual reality models allow a surgeon to preoperatively practice VATS procedures in a virtual environment and can be one the most promising simulation tools in risk-free based teaching platforms.
Conclusions
As long as the developments continue to be made in the field of surgical training and education for the coming generation of thoracic surgeons, further development of surgical simulation can continue. Although much has been described about the need of a balanced training in general, cardiac, open thoracotomy, laparoscopic surgery, and VATS, limitations in work hours and increased concerns for patient safety resulted in decreased clinical exposure and increased off-patient practice in surgical training. Simulation based practice might be able to accelerate procedural progress relating VATS with the learning curve. There are possible concerns that surgeons could lose the bigger picture, misunderstand a complex reality as the oversimplification, and become fixated on events in the narrow operating field to the ignorance of wider concerns. We need to consider how structured and formal VATS training platform incorporating with surgical simulations should be built for the new generations of surgeons and hope that not only multiportal VATS but also uniportal VATS and robot-assisted surgery will be widely and safely distributed into many medical facilities in the future for saving a large number of patient’s lives.
Acknowledgments
Funding: None.
Footnote
Provenance and Peer Review: This article was commissioned by the editorial office, Video-Assisted Thoracic Surgery for the series “Current Status of Surgical Simulation in Video-assisted Thoracic Surgery and Robot-assisted Thoracic Surgery”. The article has undergone external peer review.
Conflicts of Interest: The author has completed the ICMJE uniform disclosure form (available at http://dx.doi.org/10.21037/vats.2018.08.02). The series “Current Status of Surgical Simulation in Video-assisted Thoracic Surgery and Robot-assisted Thoracic Surgery” was commissioned by the editorial office without any funding or sponsorship. YS served as the unpaid Guest Editor of the series and serves as an unpaid editorial board member of Video-Assisted Thoracic Surgery from Dec 2016 to May 2019. The author has no other conflicts of interest to declare.
Ethical Statement: The author is accountable for all aspects of the work in ensuring that questions related to the accuracy or integrity of any part of the work are appropriately investigated and resolved.
Open Access Statement: This is an Open Access article distributed in accordance with the Creative Commons Attribution-NonCommercial-NoDerivs 4.0 International License (CC BY-NC-ND 4.0), which permits the non-commercial replication and distribution of the article with the strict proviso that no changes or edits are made and the original work is properly cited (including links to both the formal publication through the relevant DOI and the license). See: https://creativecommons.org/licenses/by-nc-nd/4.0/.
References
- Gung Y, Zhang H, Li S, et al. Sternotomy versus video-assisted thoracoscopic surgery for thymectomy of myasthenia gravis patients: A meta-analysis. Asian J Endosc Surg 2016;9:285-94. [Crossref] [PubMed]
- Paul S, Altorki NK, Sheng S, et al. Thoracoscopic lobectomy is associated with lower morbidity than open lobectomy: a propensity-matched analysis from the STS database. J Thorac Cardiovasc Surg 2010;139:366-78. [Crossref] [PubMed]
- Swanson SJ, Meyers BF, Gunnarsson CL, et al. Video-assisted thoracoscopic lobectomy is less costly and morbid than open lobectomy: a retrospective multiinstitutional database analysis. Ann Thorac Surg 2012;93:1027-32. [Crossref] [PubMed]
- Nwogu CE, D'Cunha J, Pang H, et al. VATS lobectomy has better perioperative outcomes than open lobectomy: CALGB 31001, an ancillary analysis of CALGB 140202 (Alliance). Ann Thorac Surg 2015;99:399-405. [Crossref] [PubMed]
- Vannucci F, Gonzalez-Rivas D. Is VATS lobectomy standard of care for operable non-small cell lung cancer? Lung Cancer 2016;100:114-9. [Crossref] [PubMed]
- Bendixen M, Jorgensen OD, Kronborg C, et al. Postoperative pain and quality of life after lobectomy via video-assisted thoracoscopic surgery or anterolateral thoracotomy for early stage lung cancer: a randomised controlled trial. Lancet Oncol 2016;17:836-44. [Crossref] [PubMed]
- Bandoh T, Shiraishi N, Yamashita Y, et al. Endoscopic surgery in Japan: The 12th national survey(2012-2013) by the Japan Society for Endoscopic Surgery. Asian J Endosc Surg 2017;10:345-53. [Crossref] [PubMed]
- Rodgers-Fischl PM, Martin JT, Saha SP. Video-Assisted Thoracoscopic versus Open Lobectomy: Costs and Outcomes. South Med J 2017;110:229-33. [Crossref] [PubMed]
- Boffa DJ, Dhamija A, Kosinski AS, et al. Fewer complications result from a video-assisted approach to anatomic resection of clinical stage I lung cancer. J Thorac Cardiovasc Surg 2014;148:637-43. [Crossref] [PubMed]
- Cao C, Manganas C, Ang SC, et al. Video-assisted thoracic surgery versus open thoracotomy for non-small cell lung cancer: a meta-analysis of propensity score-matched patients. Interact Cardiovasc Thorac Surg 2013;16:244-9. [Crossref] [PubMed]
- Cao C, Petersen RH, Yan TD. Learning curve for video-assisted thoracoscopic lobectomy. J Thorac Cardiovasc Surg 2014;147:1727. [Crossref] [PubMed]
- Chan DT, Sihoe AD, Chan S, et al. Surgical treatment for empyema thoracis: is video-assisted thoracic surgery "better" than thoracotomy? Ann Thorac Surg 2007;84:225-31. [Crossref] [PubMed]
- Cao C, Zhu ZH, Yan TD, et al. Video-assisted thoracic surgery versus open thoracotomy for non-small-cell lung cancer: a propensity score analysis based on a multi-institutional registry. Eur J Cardiothorac Surg 2013;44:849-54. [Crossref] [PubMed]
- Gonzalez-Rivas D, Fieira E, Delgado M, et al. Evolving from conventional video-assisted thoracoscopic lobectomy to uniportal: the story behind the evolution. J Thorac Dis 2014;6:S599-603. [PubMed]
- Gonzalez-Rivas D, Fieira E, Delgado M, et al. Uniportal video-assisted thoracoscopic sleeve lobectomy and other complex resections. J Thorac Dis 2014;6:S674-81. [PubMed]
- Gonzalez-Rivas D, Fieira E, de la Torre M, et al. Bronchovascular right upper lobe reconstruction by uniportal video-assisted thoracoscopic surgery. J Thorac Dis 2014;6:861-3. [PubMed]
- Parsons BA, Blencowe NS, Hollowood AD, et al. Surgical training: the impact of changes in curriculum and experience. J Surg Educ 2011;68:44-51. [Crossref] [PubMed]
- Simpson C, Cottam H, Fitzgerald JE, et al. The European working time directive has a negative impact on surgical training in the UK. Surgeon 2011;9:56-7. [Crossref] [PubMed]
- Hutter MM, Kellogg KC, Ferguson CM, et al. The impact of the 80-hour resident workweek on surgical residents and attending surgeons. Ann Surg 2006;243:864-71; discussion 871-5. [Crossref] [PubMed]
- Bradley P, Postlethwaite K. Simulation in clinical learning. Med Educ 2003;37:1-5. [Crossref] [PubMed]
- Kneebone R. Simulation in surgical training: educational issues and practical implications. Med Educ 2003;37:267-77. [Crossref] [PubMed]
- van der Meijden OA, Schijven MP. The value of haptic feedback in conventional and robot-assisted minimal invasive surgery and virtual reality training: a current review. Surg Endosc 2009;23:1180-90. [Crossref] [PubMed]
- Dosis A, Aggarwal R, Bello F, et al. Synchronized video and motion analysis for the assessment of procedures in the operating theater. Arch Surg 2005;140:293-299. [Crossref] [PubMed]
- Linsky PL, Wei B. Training in robotic thoracic surgery. J Vis Surg 2018;4:1. [Crossref] [PubMed]
- Agha RA, Fowler AJ. The role and validity of surgical simulation. Int Surg 2015;100:350-7. [Crossref] [PubMed]
- Bedetti B, Schnorr P, Schmidt J, et al. The role of wet lab in thoracic surgery. J Vis Surg 2017;3:61. [Crossref] [PubMed]
- Jimenez M, Gomez-Hernandez MT. Teaching video-assisted thoracic surgery lobectomy-using an ex vivo simulation model. J Vis Surg 2017;3:34. [Crossref] [PubMed]
- Badash I, Burtt K, Solorzano CA, et al. Innovations in surgery simulation: a review of past, current and future techniques. Ann Transl Med 2016;4:453. [Crossref] [PubMed]
- Jensen K, Ringsted C, Hansen HJ, et al. Simulation-based training for thoracoscopic lobectomy: a randomized controlled trial: virtual-reality versus black-box simulation. Surg Endosc 2014;28:1821-9. [Crossref] [PubMed]
- Tedde ML, Brito Filho F, Belmonte Ede A, et al. Video-assisted thoracoscopic surgery in swine: an animal model for thoracoscopic lobectomy training. Interact Cardiovasc Thorac Surg 2015;21:224-30. [Crossref] [PubMed]
- Jensen K, Bjerrum F, Hansen HJ, et al. A new possibility in thoracoscopic virtual reality simulation training: development and testing of a novel virtual reality simulator for video-assisted thoracoscopic surgery lobectomy. Interact Cardiovasc Thorac Surg 2015;21:420-6. [Crossref] [PubMed]
- Petersen RH, Hansen HJ. Learning curve associated with VATS lobectomy. Ann Cardiothorac Surg 2012;1:47-50. [PubMed]
- Seymour NE, Gallagher AG, Roman SA, et al. Virtual reality training improves operating room performance: results of a randomized, double-blinded study. Ann Surg 2002;236:458-63; discussion 463-4. [Crossref] [PubMed]
- Noda M, Mizuma M, Maeda S, et al. Presented at the 64th Annual Scientific Meeting of the Japanese Association for thoracic surgery: wet-lab training for thoracic surgery at the laboratory animal facilities. Gen Thorac Cardiovasc Surg 2012;60:756-9. [Crossref] [PubMed]
- Sarker SK, Patel B. Simulation and surgical training. Int J Clin Pract 2007;61:2120-5. [Crossref] [PubMed]
- Larsen CR, Soerensen JL, Grantcharov TP, et al. Effect of virtual reality training on laparoscopic surgery: randomised controlled trial. BMJ 2009;338:b1802. [Crossref] [PubMed]
- Schijven MP, Jakimowicz JJ, Broeders IA, et al. The Eindhoven laparoscopic cholecystectomy training course--improving operating room performance using virtual reality training: results from the first E.A.E.S. accredited virtual reality trainings curriculum. Surg Endosc 2005;19:1220-6. [Crossref] [PubMed]
- Chang L, Petros J, Hess DT, et al. Integrating simulation into a surgical residency program: is voluntary participation effective? Surg Endosc 2007;21:418-21. [Crossref] [PubMed]
- Valentine R, Padhye V, Wormald PJ. Simulation Training for Vascular Emergencies in Endoscopic Sinus and Skull Base Surgery. Otolaryngol Clin North Am 2016;49:877-87. [Crossref] [PubMed]
- Sutherland LM, Middleton PF, Anthony A, et al. Surgical simulation: a systematic review. Ann Surg 2006;243:291-300. [Crossref] [PubMed]
- Gurusamy KS, Aggarwal R, Palanivelu L, et al. Virtual reality training for surgical trainees in laparoscopic surgery. Cochrane Database Syst Rev 2009;CD006575 [PubMed]
- Sturm LP, Windsor JA, Cosman PH, et al. A systematic review of skills transfer after surgical simulation training. Ann Surg 2008;248:166-79. [Crossref] [PubMed]
- Ikeda N, Yoshimura A, Hagiwara M, et al. Three dimensional computed tomography lung modeling is useful in simulation and navigation of lung cancer surgery. Ann Thorac Cardiovasc Surg 2013;19:1-5. [Crossref] [PubMed]
- Saji H, Inoue T, Kato Y, et al. Virtual segmentectomy based on high-quality three-dimensional lung modelling from computed tomography images. Interact Cardiovasc Thorac Surg 2013;17:227-32. [Crossref] [PubMed]
- Hagiwara M, Shimada Y, Kato Y, et al. High-quality 3-dimensional image simulation for pulmonary lobectomy and segmentectomy: results of preoperative assessment of pulmonary vessels and short-term surgical outcomes in consecutive patients undergoing video-assisted thoracic surgerydagger. Eur J Cardiothorac Surg 2014;46:e120-6. [Crossref] [PubMed]
- Makino Y, Shimada Y, Hagiwara M, et al. Assessment of emphysema severity as measured on three-dimensional computed tomography images for predicting respiratory complications after lung surgery. Eur J Cardiothorac Surg 2018; [Epub ahead of print]. [Crossref] [PubMed]
- Eschweiler J, Stromps JP, Fischer M, et al. A biomechanical model of the wrist joint for patient-specific model guided surgical therapy: Part 2. Proc Inst Mech Eng H 2016;230:326-34. [Crossref] [PubMed]
- Eschweiler J, Stromps JP, Fischer M, et al. Development of a biomechanical model of the wrist joint for patient-specific model guided surgical therapy planning: Part 1. Proc Inst Mech Eng H 2016;230:310-25. [Crossref] [PubMed]
- Makiyama K, Yamanaka H, Ueno D, et al. Validation of a patient-specific simulator for laparoscopic renal surgery. Int J Urol 2015;22:572-6. [Crossref] [PubMed]
Cite this article as: Shimada Y. Future strategy of surgical simulations in video-assisted thoracic surgery. Video-assist Thorac Surg 2018;3:31.